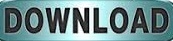
(17) The optimum TiO 2 thickness (10 nm) maximizes the open-circuit voltage (see discussion below) and minimizes resistive losses. For all devices, the thickness of the ITO layer was adjusted according to the thickness of the TiO 2 layer to maintain a total oxide thickness of ∼70 nm, such that the reflectance minimum falls in the spectral range where the solar spectrum is strongest (around a wavelength of 600 nm) in order to maximize light in-coupling into the InP absorber.
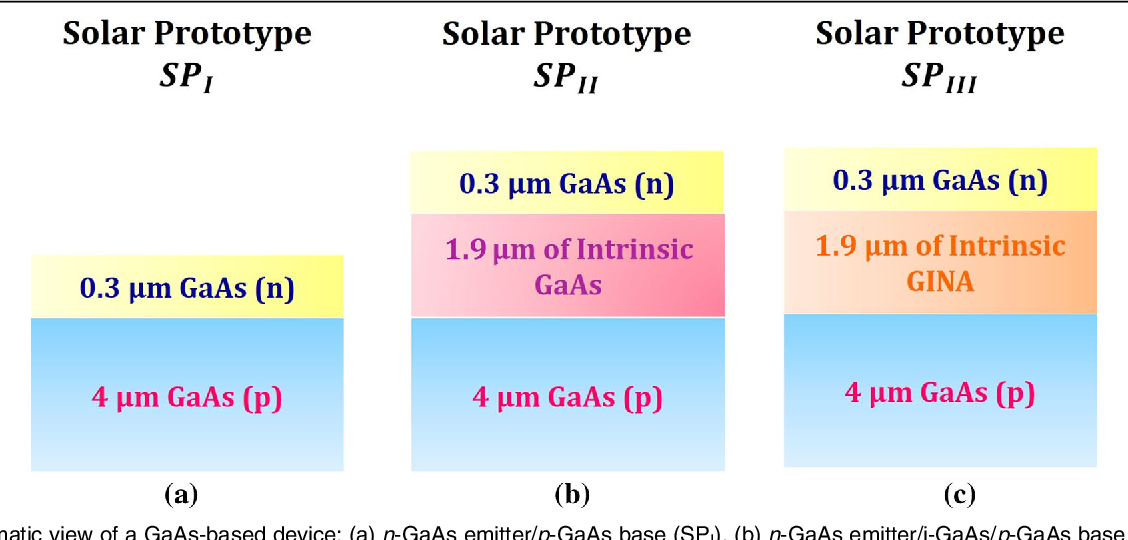
Finally, a transparent conductive oxide (ITO = In 2O 3:SnO = 90%:10%) electrode is sputtered on top of the TiO 2 layer at room temperature in Ar ambient at a pressure of 6–7 mTorr. TiO 2 is subsequently deposited onto the front side of the InP wafers by ALD using titanium isopropoxide (Ti 4) and H 2O as precursors. Twenty nanometers of Zn followed by 100 nm of Au are then sputtered onto the back side of the InP wafers, followed by annealing in forming gas (5% H 2, 95% N 2) at 420 ☌ for 40 min to form an ohmic back contact. Zn-doped p-type (100)-oriented InP wafers with a thickness of 350 μm and a carrier density of 2 × 10 17 cm –3 are etched in HCl (6%) for 30 s to remove the native oxide. The process flow for fabricating TiO 2/InP solar cells is shown in Figure 1a. (23, 24) Here, by employing a TiO 2 electron contact deposited by atomic layer deposition (ALD) at a low temperature of 120 ☌, we demonstrate a TiO 2/InP heterojunction solar cell with a high open-circuit voltage of 785 mV and an excellent efficiency of 19.2%. (22) In another approach, a CdS window layer is used with InP to form a heterojunction solar cell with an efficiency of 17.4%. This approach yields efficiencies of up to 18.9%. (21) Alternatively, a shallow buried homojunction can be formed in InP by sputtering indium tin oxide (ITO) on the front surface. (20) The most efficient InP solar cells use a homojunction configuration fabricated by metal–organic vapor deposition (MOCVD), reaching efficiencies of up to 22.1%. We focus in particular on InP, whose band gap of 1.35 eV lies close to the optimum for solar energy conversion. In this work, we show that transition metal oxides can also be applied as selective contacts for III–V solar cells. (17, 18) Analogously, TiO 2 has been used as an electron-selective contact to p-type silicon.
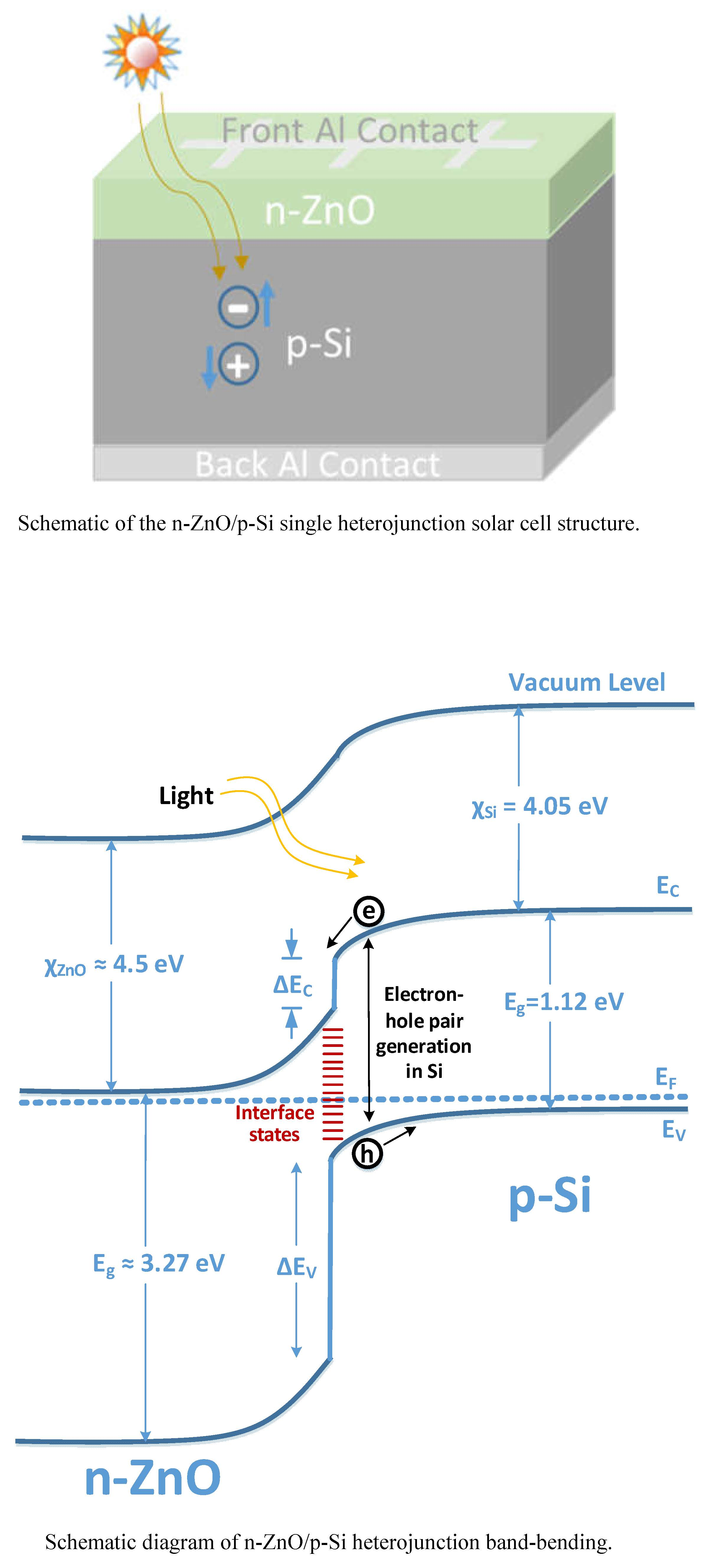
(4) Recently we demonstrated that MoO x can also serve as an efficient hole contact for n-type silicon solar cells, enabling a high open-circuit voltage of 711 mV and an efficiency of 18.8%. (2-8) In particular TiO 2 is applied widely as an electron contact in dye-sensitized solar cells, (9, 10) polymer solar cells, (11, 12) and high-performance organometallic perovskite solar cells, (13-16) while substoichiometric MoO x ( x < 3) has emerged as an excellent hole contact. For organic solar cells, in contrast, transition metal oxides have proven to be efficient selective contacts. (1) Traditionally inorganic semiconductors use doping to achieve carrier selectivity at the contacts. Selective contacts that transmit photogenerated minority carriers and block majority carriers are key to high-performance solar cells.
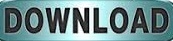